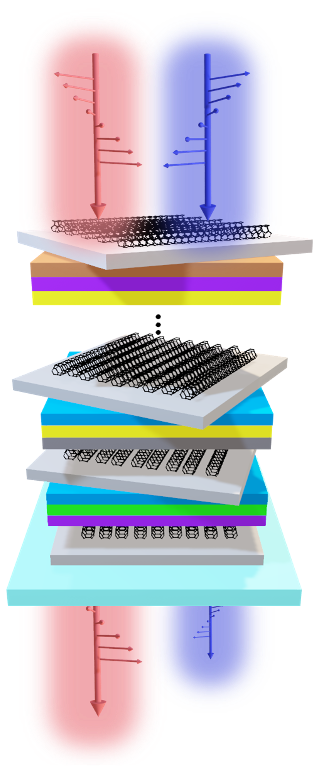
As fast as modern electronics have become, they could be much faster if their operations were based on light, rather than electricity. Fiber optic cables already transport information at the speed of light; to do computations on that information without translating it back to electric signals will require a host of new optical components.
Researchers at the John and Marcia Price College of Engineering have now developed such a device: one that can be adjusted on the fly to give light different degrees of circular polarization. Because information can be stored in this chiral property of light, the researchers’ device could serve as a multifunctional, reconfigurable component of an optical computing system.
Led by Weilu Gao, assistant professor in the Department of Electrical & Computer Engineering, and Jichao Fan, a Ph.D. candidate in his lab, a study demonstrating the device was published in the journal Nature Communications. Fellow Gao lab members Ruiyang Chen, Minhan Lou, Haoyu Xie, Benjamin Hillam, Jacques Doumani, and Yingheng Tang contributed to the study, as did Nina Hong of the J.A. Woollam Company.
“Traditional chiral optics were like carved stone—beautiful but frozen,” says Gao. “This made them not useful for applications requiring real-time control, like reconfigurable optical computing or adaptive sensors.”
“We’ve created ‘living’ optical matter that evolves with electrical pulses,” says Fan, “thanks to our aligned-carbon-nanotube-phase-change-material heterostructure that merges light manipulation and memory into a single scalable platform.”
This “heterostructure” consists of a stack of multiple different thin films, including a collection of aligned carbon nanotubes with different orientations. Other films in the stack consist of GST, a well-known “phase-change material” or PCM. An electrical pulse along the carbon nanotube layer introduces heat, which in turn causes the PCM layer’s internal structure to transition from amorphous to crystalline.
“The carbon nanotubes simultaneously act as chiral optical elements and transparent electrodes for PCM switching—eliminating the need for separate control components,” says Fan.
Critically, this change modifies the heterostructure’s circular dichroism, which means it can be made to absorb different types of circularly polarized light at different strengths. The research team’s advances in manufacturing techniques and artificial-intelligence-assisted design enabled these layers to be assembled into a stacked heterostructure without degrading their individual optical properties.
Once assembled, the layers selectively reduce the amount of left- or right-circularly polarized light that passes through them, depending on the state of the PCM layer. And because that phase change can be initiated by an electrical pulse, the structure’s overall circular dichroism can be adjusted in real-time.
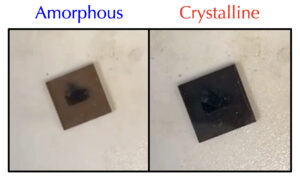
The researchers were able to achieve this on the wafer-scale, because of the scalable manufacturing of aligned carbon nanotubes and phase-change-material films.
Being able to modify the device’s circular dichroism gives researchers fine-grained control over which direction circularly polarized light twists, meaning its “handedness” can be used as memory in an optical circuit. In addition to light’s speed advantage over electricity, there are additional properties of light in which information can be stored in parallel.
“By adding circular dichroism as an independent parameter, we create an orthogonal information channel,” says Gao. “Adjusting it does not interfere with other properties like amplitude or wavelength.
The research was supported by the National Science Foundation through Grants No. 2230727, No. 2235276, No. 2316627, and No. 2321366.