All the way at the bottom of the Periodic Table, tucked away in an inset, you’ll find a double row of unusual elements: the actinides and lanthanides. Some of these metals are abundant in the Earth’s crust, while others can only exist for split-seconds after being synthesized in advanced labs, but the chemical properties that define them are indispensable for a host of modern technologies.
Tara Mastren, assistant professor in the Nuclear Engineering Program housed in the Department of Civil & Environmental Engineering, specializes in this chemistry and its applications at the intersection of nuclear science and medicine. Various diagnostics and radiotherapies need specific isotopes of these elements, but as they are all inherently unstable, researchers like Mastren devise ways to capture them in their most useful states.
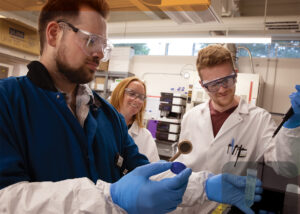
Mastren’s lab doesn’t just produce rare isotopes, however. It produces expertise.
Two of Mastren’s students, Aidan Bender and Connor Holiski, were selected to participate in the U.S. Department of Energy’s Office of Science Graduate Student Research (SCGSR) program. Each year, this DOE program places graduate students into National Laboratories, giving them access to state-of-the-art equipment to continue their research and share knowledge with experts in their fields.
Bender and Holiski are among 87 students in this year’s class, and the only two from Utah.
“This is an extremely prestigious program,” says Mastren, “and I couldn’t be prouder of Aidan and Connor.”
The field of nuclear medicine involves using specific radioactive isotopes to destroy cancer cells or as part of advanced imaging technologies. These isotopes are carefully chosen based on the types of radiation they emit; different energetic particles can last longer or travel farther than others, making the selection for a given application a matter of both efficacy and safety.
Selecting the right isotope is one challenge; acquiring enough of it is another. The actinides, which include uranium and plutonium, are radioactive but many of them are rarely found in nature. The lanthanides, despite their moniker of “rare-earth metals,” are much more common, but aren’t naturally radioactive. As such, they must first be “transmuted” into the radioactive atoms called radionuclides via nuclear reactions. Mastren’s research involves investigating such nuclear reactions using the University of Utah’s TRIGA reactor.
Once transmuted into a radionuclide, the atoms begin to decay, emitting alpha particles or electrons at a predictable rate. Each step of decay makes a new radionuclide — so timing is critical when it comes to capturing the material in its desired state.
Bender and Holiski work on different aspects of this process.
Holiski’s research is in creating resins that can capture terbium-161, a radioisotope of the lanthanide terbium that is used in both cancer treatments and diagnostic imaging.
Different lanthanides are very difficult to separate from one another; Holiski’s new resins are designed to be more effective at isolating them. And as radio-lanthanides are also a byproduct of the fuel cycle used in power-generating nuclear reactors, Holiski will spend six months continuing his resin research at Lawrence Livermore National Labs, exploring whether his technique can be used to mitigate radioactive waste.
“Tara has been a great mentor, not just because of the knowledge she passes on, but because she is always looking out for these sorts of opportunities and pushing us to apply to them,” says Holiski.
Bender’s work is on the synthesis of multimodal chelates, ringshaped molecules that can bind both radionuclides and the biomolecules that are used for delivering them to only cancerous cells. Such precision is necessary to identify the microscopic tumors treated by targeted alpha-therapy, which is highly effective but comes with the risk of destroying healthy cells if the cancer cells aren’t targeted efficiently.
Mastren’s lab has been collaborating with the Idaho National Laboratory in the creation of a new class of chelates; Bender will continue his work there this fall.
“The National Lab environment is exciting because there are so many people in so many disciplines,” Bender says. “It brings together materials science, fusion research, and basic biology.”
As the Mastren Lab’s family tree continues to extend its branches into these other topics and laboratories, the benefits flow in both directions.
“Nuclear medicine is inherently interdisciplinary,” says Mastren, “so building up these networks is fundamental to our work.”