Orderly, engineered artificial systems, whether they’re constructed out of bits, atoms, electronic circuitry, or mechanical gears, seem to stand in stark contrast with the squishy, chaotic world of cells, proteins, and nucleic acids that comprise natural biological systems.
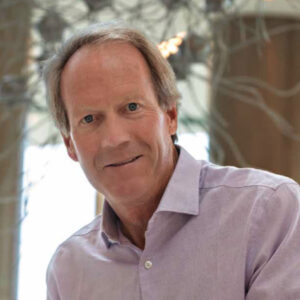
For David Grainger, chair of the Department of Biomedical Engineering (BME), however, the barrier separating those two worlds is becoming increasingly diffuse. This technological overlap is endemic to the biomedical engineering field; roughly half of BME students perform their research in conjunction with School of Medicine and Health Sciences partners, and multiple BME faculty are cross-appointed into these departments. And significantly, more parts of the campus are forging inroads to biomedical engineering than ever before.
“Opportunities within healthcare to produce knowledge, education, and new technologies are expanding constantly, and involving increasingly diverse expertise and departments,” Grainger says. “That’s inspired a lot of students and faculty to get involved with our department, and we’re carrying that momentum forward with broader research and new educational offerings.”
As with many revolutions, scientific or otherwise, a crisis precipitated this change. The COVID pandemic thrust biomedical engineers onto the frontlines of a global battle with life-or-death stakes. Lacking much clinical validation for decades, the technology behind mRNA-based vaccines was revitalized in a crash program, with researchers turning a proof-of-concept into 13 billion effective doses in less than three years. This urgency also promoted interdisciplinary collaborations responsible for other innovations; materials scientists and mechanical engineers tapped BME expertise to load mRNA into safe delivery systems, develop personal protective equipment and patient mechanical ventilators, and innovate instant PCR testing methods.
Of course, the next revolution doesn’t wait for the last one to finish; BME routinely responds to new challenges with partnered interdisciplinary design teams that address complex healthcare technology ambitions. As soon as “machine learning” and “big data” became commonplace terms within the discipline, biomedical engineers were forced to wrangle with new opportunities and applications. Targeted to sift through impossibly large sets of chemical permutations in search of drug candidates; automatically identify critical features in biomarker disease profiles and human-scale diagnostic imaging; or flag clinical features in patient electronic health records, new algorithmic technologies show great promise — and potential peril.
The decisions that AI-based tools make are only as good as the data upon which they are trained, and still must be implemented and verified by human clinicians on human patients. As such, the department is collaborating with other campus schools, institutes, and departments, as well as University-wide initiatives like DELPHI — Data Exploration and Learning for Precision Health Intelligence — to provide a path forward.
“We’re in active discussions with our colleagues in the Kahlert School of Computing, Medicine’s Department of Bioinformatics, Hunstman Cancer Institute, UCAIR, and others, to help guide how we train our students to engage with data science and AI as they evolve in healthcare technology,” Grainger says. “These technologies, relatively new to BME endeavors, will alter future aspects of the BME discipline. As Chair, I’d prefer to act on our mission now than react later.”
In many cases, that future is already here; new subfields where the biological, the biomedical and the technical merge to inform and complement one another are going viral. Recent advances in synthetic biology allow genetic sequences and their functional operations to be cut-and-pasted like lines of code between different organisms. Neuroengineering researchers are developing a new, innovative brain- machine interfaces, in which synaptic signals are directly read and translated into digital messages, which can then be deciphered and converted into action. This microelectronic communication conduit into tissue can mitigate the effects of stroke or spinal cord injuries, even allow patients to control computer interfaces and robotic operations with a thought.
On a smaller scale, wearables that record heart rates, sleep cycles, and other biometrics, will digitally monitor increasingly diverse physiological and pathological indicators enabling better telehealth and remote medical interfacing.
“The limited biometric reporting of your Apple Watch is just the tip of the iceberg,” says Grainger. “The improved capabilities and fidelity of these sensors, their validation with regulatory bodies, and the rise of telemedicine in general, are rapidly improving medical technologies that provide physicians access to types, formats and amounts of health data we’ve never had before. This real-time remote monitoring capability will produce a dramatic shift in how patients are handled in the healthcare system.”
Huge amounts of patient-derived medical data are also helping biological and computational technologies merge in an even more comprehensive way, through the concept of a “digital twin.”
“If we had enough reliable, quality data to inform how the complex, interactive and often chaotic physiological processes in our bodies work to produce both health and disease, we could simulate them with elaborate computational models,” Grainger says. “Then we wouldn’t need to rely on mouse or zebrafish models to mimic human conditions, or blindly tweak a black-box experimental model to merely approximate what’s going on inside a specific patient.”
As these revolutionary technologies take further shape and disrupt the landscape of current healthcare protocols, Grainger sees anticipating and harnessing such changes as being inherent to his department’s mission in research, training, and teaching.
“Our department has always focused on the value derived from medicine and engineering working together to address unmet clinical and healthcare needs,” Grainger says. “BME relies on partnerships, teaming, and inter-personal engagement to perform our mission. It’s necessary because of the breadth and complexity of human healthcare, rapid advances in both engineering capabilities and clinical standards of care, and evolving clinician needs in better addressing patients.”
“Ultimately,” he says, “we’re a melting pot of faculty, students and disciplines, breaking down traditional academic silos in pursuit of that interdisciplinary and translational biomedical mission.”